Personal trainers who continue to develop a growing knowledge of the exercise sciences are able to communicate effectively with established health professionals and sciences on “common ground” and also apply such knowledge in expert client programming. While the following is quite technical, it provides an integral part of the knowledge base necessary to facilitate such communication and skill development. Read on to explore how human movement science can help you to become a better personal trainer.
The Complexity of Biomechanics and Human Movement Science
Physical education has evolved in recent years from physical training and coaching to kinesiology, the study of movement. To the general public, this change has gone unnoticed. For those in the fitness professions, kinesiology may seem mysterious. Kinesiology includes a broad range of disciplines such as exercise physiology, sport psychology, sport sociology, motor control, and biomechanics.
Biomechanics
Biomechanics is the science that applies the laws of mechanics to biological movement. One area of interest to biomechanists is studying the body during sport or exercise situations.
Sports biomechanists focus on two fundamental concepts: how to improve performance and how to make activities safer. Two sub-disciplines of biomechanics address these concepts:
1) kinematics: the description of motion
2) kinetics: the study of the forces that act on the body.
Kinematics and Kinetics Defined
Biomechanists use kinematics to analyze the motion of a skilled athlete. The assumption is that the skilled athlete has learned the most efficient way to perform the movement, and that others can improve simply by copying the successful athlete. Kinetics is employed to find a more efficient way of moving, making better use of the mechanical advantage of the body.
Kinematics and kinetics are used to determine the forces acting on the body, identifying ways to decrease the forces that cause injury by altering the movement or designing equipment that would reduce the forces acting on the body. One example of the latter approach is the improvement of shoe design in recent years.
Applying the basic tools described above, sports biomechanists are able to examine some problems of interest in sports and exercise. In order for the research performed by the biomechanists to benefit those who need it, there needs to be more communication between all exercise scientists, including biomechanists, and the fitness professionals who are in a position to apply their research. Like many of the disciplines of kinesiology, biomechanists need the input of practitioners for suggesting future research.
Kinematics
In kinematics, the limbs or segments of the body are assumed to rotate about the joints, with no translational, or sliding, movement. While this is not strictly correct, it offers a usable approximation of the actual joint motion. The joint serves as an axis (a line around which something can rotate), and associated with the axis is a plane, (like a sheet of paper perpendicular to the axis), in which the rotational movement takes place.
Borrowing from mathematics, the location of the body is defined in a three-dimensional coordinate system. Using this system and anatomical position, the three axes and their associated planes are defined.
The anatomic position is important in defining several concepts of location with respect to the body:
Proximal: Segment closer to the head
Distal: Segment farthest from the head
Medial: Closest to midline of the body
Lateral: Farthest from midline of the body
Anterior: Segment located at front of the body
Posterior: Segment located at rear of the body
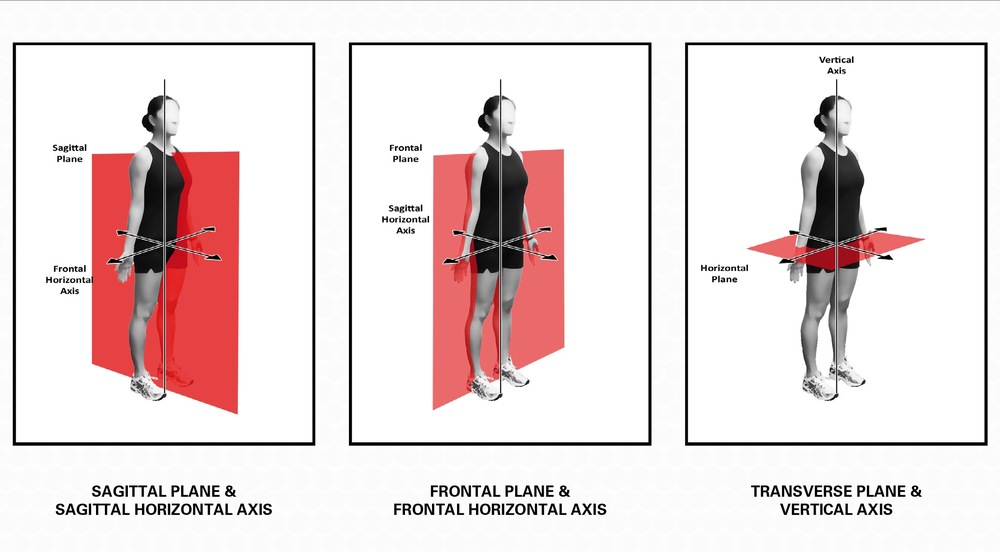
Photo credit: perfectstridept.com
The sagittal plane divides the body into left and right halves. The mediolateral axis (also known as the frontal axis) runs side-to-side at a right angle to the sagittal plane. The primary movements in the sagittal plane are flexion and extension.
- Flexion occurs when the distal end of the segment is moved forward as in bending the elbow
- Extension occurs when the distal end of the segment is returned to the anatomic position as in straightening the elbow.
- Hyperextension can occur when the distal end of the segment is moved toward the posterior side of the body.
The anteroposterior axis (or sagittal axis) runs from front to back, intersecting with the front plane at a right angle. Movements around the anteroposterior axis are said to occur in the frontal plane, which separates the anterior and posterior sides. The primary movements in the frontal plane are abduction and adduction.
- Abduction occurs when the distal end of a segment moves laterally (away from the body) as in raising your arm out to your side (shoulder abduction).
- Adduction occurs as the distal end of the segment moves medially as in returning your arm back to your side (shoulder adduction).
The third axis is called the longitudinal axis, which runs from the top to the bottom of the body. Rotations around the longitudinal axis take place in the transverse or horizontal plane. The primary movements in the transverse plane are medial and lateral rotation.
- Medial rotation (often referred to as internal rotation) occurs when the lateral aspect of the segment moves towards the midline of the body, rotating first to the anterior side such as turning the arm inward towards the chest, palm facing backward.
- Lateral rotation (often referred to as external rotation)(occurs when the rotation occurs in the opposite direction; the medial aspect of the segment rotates toward the posterior side such as turning the arm outward and palm forward.
It should be noted that the actions at the joints are always described as if the body were in the anatomic position. For example, if you abduct the shoulder, the elbow is moved into a position where the elbow, if flexed, technically would be doing so in the transverse plane (as in starting a bench press which does indeed take place in the transverse plane).
By disregarding how the elbow flexes in the anatomic position, this action of the elbow might be construed as medial rotation rather than flexion. However, we know the elbow flexes and extends in the anatomic position—a motion that occurs in the sagittal plane–and so that’s the action we will always assign to the elbow.
While the primary motions about the axis have been described above, a few joints have different designations for their motions about the axis. For example, the big toe moving toward the midline of the body is called abduction because it’s moving away from the midline of the foot–its main point of reference–not the entire body as is usually the point of reference.
The position of the body in three dimensions can be described using a combination of movements at all of the joints, and moving around all three axes. In addition, the speed of motion around the joints, or angular velocity, and the change in velocity, or angular acceleration can be examined, usually by recording a subject performing the motion. The velocity and acceleration can also be identified by using kinetics, as is described in the next section.
We also note the difference between open-chain and closed-chain movements concerning biomechanics. In an open-chain movement, the hand or the foot is not in contact with a surface, and is therefore free to move under the resistance. Think, bicep curl or leg extension. A closed-chain movement keeps the hand or foot stabilized against a surface, as in a lunge or a push-up.
Kinetics
Kinematics, while useful in describing motion, tells us nothing about the forces which created that movement. To examine the forces acting on the body, kinetics involves examining external resistance, gravity, and muscle forces using the laws of mechanics discovered by Sir Isaac Newton in the 1600s.
The forces acting on the body are divided into two categories: external and internal forces. External forces arise from outside the body. Gravity is one example: a pull exerted by the earth on the body. Other examples include wind resistance and the use of outside weights to create resistance.
Internal forces are those which arise from inside the body and include muscle forces, the forces exerted by ligaments, and bone-on-bone forces. In order to create a movement, internal forces must be generated in the proper degree to overcome the external forces acting on the body. Every force has four qualities:
1) magnitude, or quantity of force
2) direction
3) point of application of the force
4) a line of action indicating where the force is going.
The force accelerates the segment (limb), changing either the speed or direction of the segment, along the line of action of the force according to Newton’s second law of motion:
F=ma
where F is the force applied, m is the mass of the segment, and a is the acceleration.
The description of muscular contractions is based on the motion of the segment. If the segment is not moving and the muscle is contracting, the contraction is called an isometric contraction. If the system is such that the segment is moving in the direction of the muscle force, the muscle is said to be going through a concentric contraction. If the segment is moving away from the direction of the muscle force, the muscle is engaged in an eccentric contraction.
If the force does not act directly on a joint it is called an eccentric, or off-center, force. An eccentric force, in addition to accelerating the body or limb it is acting upon, will also cause the body or limb to rotate about its axis. The rotating force is called a moment of force, sometimes referred to as torque.
The moment of force, or simply the moment, about each axis has two properties: magnitude and direction, which can be positive or negative. By convention, a moment that causes a clockwise motion is called negative, while counterclockwise moment is called positive. The magnitude of the moment is determined by the equation:
M=Fd
M is the magnitude of the torque, F is the force applied, and d is called the moment arm.
The moment arm is equal to the shortest distance from the axis (joint) to the force acting on the limb, determined by the line of action of the force.
The moment arm is calculated using the equation: d=rsinq where r is the distance from the axis (labeled 0) to the point of application of the force and q is the angle between the segment and the force. Only in rare cases does only one force, and therefore, only one moment act on a segment.
A typical case occurs when the force of the biceps, the weight of the forearm and hand, and the weight of a dumbbell, together referred to as FR(Resistance), are acting on lifting the forearm and hand. When more than one force is acting on a limb, the moments are added together to determine the total moment acting on the limb.
The actual moment arm of the biceps muscle (MM) is small when compared to the moment arms of the other two forces. This is the situation that normally occurs in the body. A comparison of the moment arms of the forces is made when mechanical efficiency is calculated. The mechanical advantage of a system is:
Mechanical Advantage=IA/EA
where IA is the moment arm of the internal forces and EA is the moment arm of the external forces.
The acceleration of the segment depends on the moment of the system. The segment is accelerated in the direction (positive or negative) of the moment of the system: if the moment is positive, the segment is accelerated in a counterclockwise direction. The magnitude of the acceleration is given by:
M=Ia
where a is the angular acceleration of the segment and I is the moment of inertia, which represents the weight distribution of the segment.
Since the moment arms of the muscles in the body (internal forces) tend to be smaller than the moment arms of the external forces, the mechanical advantage is usually less than 1. The small mechanical advantage means that large forces must be applied to overcome the external forces. Despite this disadvantage, the small mechanical advantage is still helpful since it has the property of allowing a large range of motion for small amounts of muscle shortening.
Biomechanics and Personal Training
While some of the above information does not seem directly applicable to the development of a standard fitness program, the principles presented comprise the underlying basis of all movements performed in sport, exercise, and daily activities. Knowing when to use full or partial range of motion in particular exercises is one way to begin considering the role of human movement science and biomechanics in your practice.
Understanding the basics of these complex concepts may help personal trainers effectively apply research in program designs to favor progress and minimize injury as well as communicate the results of such implementation appropriately.
[sc name=”anatomy” ][/sc]
References
Adrian, M. and Cooper, J. The Biomechanics of Human Movement. Indianapolis, Benchmark Press, Inc., 1989.
Alexander, R.M. The Human Machine. New York, Columbia University Press, 1992.
Beer, F. and Johnston, E.R. Vector Mechanics for Engineers: Statics and Dynamics. New York, McGraw-Hill, 1988.
Kreighbaum, E. and Barthels, K.M. Biomechanics: A Qualitative Approach for Studying Human Movement. New York, Macmillan Publishing Company, 1985.
Norkin, C. and Levangie, P. Joint Structure and Function: A Comprehensive Analysis. Philadelphia, F.A. Davis Company, 1992.
Spence, A. and Mason, E. Human Anatomy and Physiology. Menlo Park, Benjamin/Cummings, 1987.
NFPT Publisher Michele G Rogers, MA, NFPT-CPT and EBFA Barefoot Training Specialist manages and coordinates educational blogs and social media content for NFPT, as well as NFPT exam development. She’s been a personal trainer and health coach for over 20 years fueled by a lifetime passion for all things health and fitness. Her mission is to raise kinesthetic awareness and nurture a mind-body connection, helping people achieve a higher state of health and wellness. After battling and conquering chronic back pain and becoming a parent, Michele aims her training approach to emphasize fluidity of movement, corrective exercise, and pain resolution. She holds a master’s degree in Applied Health Psychology from Northern Arizona University. Follow Michele on Instagram.